Failure Analysis Plays Key Role in Design and Manufacturing
Advanced Metallurgy Testing Can Identify and Resolve Issues Early
Overview
The majority of today’s product manufacturing scenarios involve metal — either in the products themselves and/or the tooling used to create them. Unsurprisingly, metallurgy-based failure analysis is an important consideration for assuring both product quality and production efficiency. Defects in end products can very quickly be detrimental to a company’s reputation and drive up the costs of subsequent product support. Defects in production tooling can impair efficiency, cause work stoppages and drive up manufacturing costs.
This Tech Bulletin provides a brief overview of metallurgy-based failure analysis and sheds light on where and how these important analyses help product manufacturers assure high-quality production output and high-efficiency production processes.
What is Metallurgy Failure Analysis
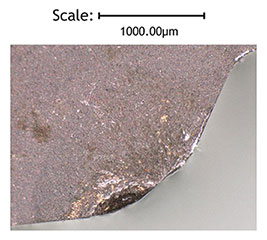
Metallurgy is an arena of materials science and engineering that focuses on physical and chemical aspects of metallic elements, inter-metallic compounds and alloys. Ferrous metallurgy covers the study of iron and iron-based alloys, while non-ferrous metallurgy encompasses all other metals.
Metallurgy-based failure analysis involves applying specialized materials science disciplines to provide an understanding of why product or tooling failures have occurred. The objective of this is to use this knowledge to develop solutions to mitigate or eliminate the conditions that led to the failures, in order to prevent them from reoccurring.
Proper failure analysis is enabling and valuable in numerous ways:
- Finding and correcting quality problems with production output
- Analyzing field failures to target the root causes and prevent them from reoccurring
- Prolonging the life of tooling, dies and punches
- Providing feedback to improve the design of new products
- Improving Design for Manufacturability and Design for Serviceability
Capabilities of Metallurgy Failure Analysis Laboratories
As covered in subsequent sections, effective failure analysis requires competent expertise a controlled laboratory environment that can apply a variety of inspection and analysis techniques. Some of these key techniques include:
- Digital Optical Microscopy
- Tensile Strength Testing
- Microhardness Testing
- Corrosion Testing
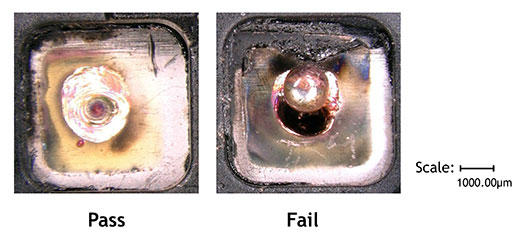
In addition to testing capabilities, the failure analysis laboratory should also have reliable processes in place for the preparation of test samples. These include procedures such as cutting, mounting, grinding and polishing prior to examination. Accountability is also of utmost importance; the laboratory must have disciplined and well-documented procedures for sample handling, tracking and reporting of results.
The following sections provide details on some of the key processes in and applications of metallurgy-based failure analysis.
Digital Optical Microscopy
Digital Optical Microscopy uses a microscope with an integrated digital camera that enables observation and analysis of the microscopic image using an attached computer. This allows for a broader range of analytic processes, and also faster overall analysis. A vibration isolation workstation should be used to ensure that the sharpest optical pictures can be obtained.
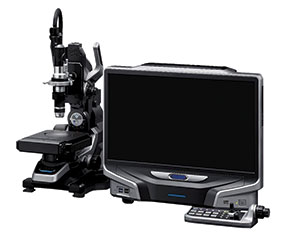
Digital Optical Microscopy enables better decisions to be made regarding material selection for products as well as tooling. It enables detailed views of surfaces as well as microstructure analysis of the material. This is useful for the observance of surface contamination and surface coating defects such as blistering of electroplated or organic coatings.
Depending on the metallurgical property being observed, samples may need to be mounted and polished before being analyzed through a digital optical microscope. The areas of observation on the parts must be sectioned to the correct size for mounting using precision cutting equipment. The cutting process should cause the least amount of thermal and mechanical damage to the samples being examined.

Takes Place After Cutting

Mounting a sample protects fragile or coated samples during preparation. Grinding (and polishing) planarizes the sample and removes any damage that results from cutting. If a surface defect is being analyzed— such as surface contamination or a fracture surface— mounting and grinding/polishing is not required. However, when observing properties like grain structure, the sample should be mounted and ground/polished beforehand.
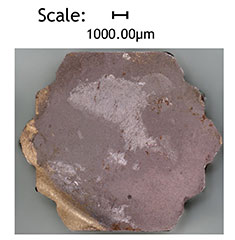
Tool Steel Punch (40X)

Tool Steel Punch (100X)
Tensile Strength Testing

Tensile Strength Testing subjects a material sample to controlled tension until failure, while measuring properties including yield strength (YS), maximum elongation, reduction in area and ultimate tensile strength (UTS).
YS is defined as the stress at which a material begins to plastically deform. UTS characterizes the capacity of loads that tend to elongate or otherwise deform the material. It determines the maximum stress that a material can withstand while being stretched or pulled before breaking.
Maximum elongation is measured as a ratio, and is representative of a material’s ductility. Some materials break very sharply — that is, without plastic deformation — in what is known as brittle failure. Other materials which are more ductile, including most metals, experience some plastic deformation and elongation before fracture.

Knowing the mechanical properties above help in choosing the right material for the right job. For example, the landing gear on long-range commercial aircraft requires the highest UTS and YS to handle the massive loads acting on the plane while parked and stationary, and also during take off and landing. The elongation of this material must be very low, such that the landing gear will not bend and kink under those same loads.
On the contrary, a rubber band has low UTS and YS because its end application does not require it to support heavy loads like an aircraft does. However, it needs a very high % elongation to accommodate stretching to hold multiple objects together.
Microhardness Testing
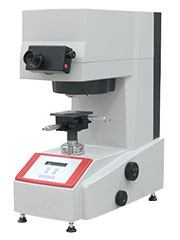
Microhardness Testing involves testing a material’s surface hardness by quantifying its resistance to deformation and indentation. Typically, the testing procedure exposes the material to an indentation process using controlled forces and the results are thereafter measured.
Microhardness Testing is critical for determining the relative hardness of materials and coatings. It is a key analysis technique used in both production quality control and helping determine up-front design choices.
Examples of applications for which Microhardness Testing can be used for include:
- Determining case hardness depth after nitriding and carburizing – these processes harden the surface to increase wear resistance, while preserving a ductile core to handle greater mechanical loads.
- Determining hardness of electroplated chrome – chrome-plating parts increases their resistance to corrosion and their wear resistance when subjected to compression or frictional loads
- Determining hardness in weld zones or heat-effected zones – for optimum corrosion resistance, and to reduce possibility of Hydrogen-embrittlement in Stainless Steel welds
- Determining hardness of sheet metal (for thicknesses <1.0mm) – to evaluate formability of sheet metal for metalworking processes
Corrosion Testing
Corrosion Testing aims to solve, prevent or mitigate corrosion-related problems. Corrosion is an electrochemical process; electrons are released by the metal (oxidation reaction) and gained by elements (reduction reaction) in the corroding solution. This flow of electrons (current) in the corrosion reaction can be measured and controlled electronically, and is the principle behind corrosion testing.

For failure analysis, electrochemistry corrosion testing is used to evaluate various materials and coatings in selected corrosive fluids at temperatures ranging from -80°C to +350°C.
Corrosion Testing is used across a wide range of industries and application environments:
- Determining the effectiveness at body temperature (37°C) of the passivation processes for Stainless Steel or Titanium parts in medical devices
- Determining the lifecycle of ceramic coatings on fuel cell plates, where operating conditions range from -80°C to + 200°C
- Evaluating anodized coatings of an aluminum part for marine use in various field conditions; its performance and longevity would differ in tropical climates versus temperate ones
Summary
Failure analysis spans across a broad domain of disciplines and methodologies. While its importance is clear, not all companies have the core competencies or bandwidth to set up a fully equipped and qualified laboratory for this purpose. For this reason, it is important to partner with a specialized laboratory that can be trusted to efficiently conduct high-quality and reliable failure analyses for the specific needs at hand.
Across a multitude of industries and a wide range of end applications, the sheer variety of products and tooling that need to be analyzed means there is no one-size-fits-all testing methodology that can be applied across the board. In many cases, a failure situation may need to undergo multiple different tests to determine the underlying causes and potential corrective actions.
Product designers and manufacturers should look toward partnering with specialists that have integrated, in-house failure analysis capabilities. This assures tight integration of such analyses as a fundamental part of their value chain, along with the ability to quickly analyze and resolve any issues that arise.
For More Information
For more information, drop us an email at communications@interplex.com.